by Nicole Gadda
Technique Name: Fluorescence In Situ Hybridization (FISH)
Fun rating: 4/5
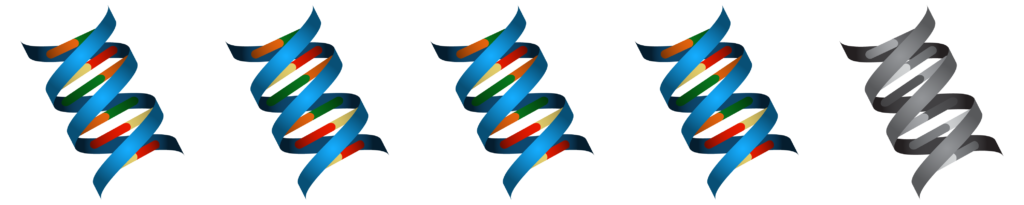
Personally, I love anything that is fluorescent (lights up) underneath a black light. I’d wear neon glow stick necklaces and bracelets daily if I could. So, when it comes to visualizing science under a fluorescent microscope, I’m hooked like a fish on a line. Therefore, I’m giving FISH a 4 out of 5 on the Fun Scale.
Difficulty rating: 4/5

What is the general purpose? I know what you’re thinking, but no, FISH isn’t a technique for making sushi rolls or frying seafood. FISH is an acronym for fluorescence in situ hybridization – a scientific technique used to detect DNA sequences inside of cells.
Why do we use it? In the past, FISH was used to map genes on the human chromosome (which can be found in a public database called the Human Genome Project). Currently, FISH is used by clinicians to diagnose diseases that result from chromosomal deletions and duplications, like Edward’s Syndrome and Down’s Syndrome. It can also be used to detect cancer and diagnose infections caused by bacteria. In addition to its clinical applications, FISH is also used by scientists to study genes of interest in their research.
How does it work? Similar to looking for a needle in a haystack, locating a single DNA sequence in a set of chromosomes can be incredibly difficult (each human cell has approximately 6 feet of DNA, and humans are estimated to be made up of 10 trillion cells!). This search is easier if you have a magnet that can draw out the needle. In FISH, the “magnet” is a fluorescent copy of the DNA sequence of interest, which we call a probe. Hybridization occurs when the “magnet” (the fluorescent copy of the DNA sequence of interest or probe) meets the “needle” (the single DNA sequence inside a cell).
The DNA in our cells consist of a double helix, or two strands, of DNA. Each strand contains a sequence of the four bases: adenine (A), guanine (G), cytosine (C), and thymine (T). Each base in a strand pairs to its complementary base on the other strand: A pairs with T, and C pairs with G. The bond with which each complementary base binds to its corresponding partner (A-T and C-G) is called hybridization.
The first step in this process is to design a probe that will hybridize with your DNA sequence of interest. To do this, it’s important to understand some fundamentals about DNA. The DNA in our cells are made of a double helix, or two strands, of DNA. Each strand contains a sequence of the four bases: adenine (A), guanine (G), cytosine (C), and thymine (T). Each base in a strand pairs to its complementary base on the other strand: A pairs with T, and C pairs with G. The bond with which each complementary base binds to its corresponding partner (A-T and C-G) is called hybridization. For example, if your target DNA sequence is “ATTGC,” then your complementary probe should be designed as “TAACG.”
Next, you have to ensure your probe sequence can fluoresce. This can be done by adding a fluorescent label (or tag) to either end of the probe sequence. Before hybridization can occur, both the target and the probe sequence must be denatured, or broken down, with heat and chemicals. Cells are fixed with paraformaldehyde – this adheres cells in place and sticks them to a microscope slide. More chemicals are used to poke holes in the membrane of cells, or break down the cell walls of bacteria, in order to ensure the probe has a way to enter the cell. The probe and cells are then mixed together, and the probe is able to get inside the cell and hybridize to the target sequence on the chromosome. The hybrid can then be visualized using a fluorescent microscope – and voila, you get beautiful, neon-colored images!
