by Nila Pazhayam
Technique Name: Mapping genetic recombination during meiosis
Fun Rating: 5/5
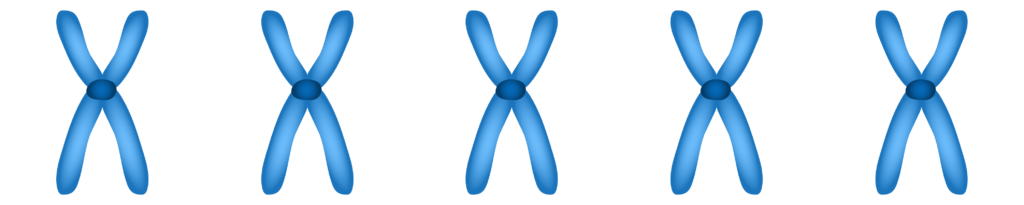
Difficulty Rating: 3/5
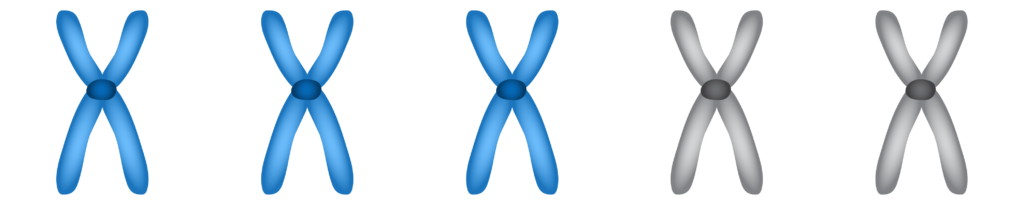
Intro: There are two types of cell division that occur in our bodies – mitosis and meiosis. A cell that divides through mitosis creates two daughter cells that are identical to the parent cell in every way, specifically the number of chromosomes present in them. This is how every cell in our body divides, with two notable exceptions: the germ cells that produce gametes (sperm cells in males and egg cells in females). Division is a little different here as these are the cells that will go on to fuse (in a process called fertilization) to produce the zygote, which later develops into the embryo and eventually the individual. If sperm and egg cells had the same number of chromosomes as a regular cell, the zygote would then have double the number of chromosomes that it should. To avoid this problem, germ cells do a clever type of cell division known as meiosis, during which they halve the number of chromosomes in sperm and egg cells before they fuse together. This ensures that the fused cell has the exact number of chromosomes as our somatic (non-germ) cells.
Another important factor that sets meiosis apart from mitosis is a process known as crossing-over – or recombination – that happens in one of the earlier steps. Cells have two copies of each chromosome, one from the mother (known as the maternal homolog) and one from the father (known as the paternal homolog). During crossing-over, there is exchange of chromosome arms between the maternal and paternal homologs of each chromosome, which causes a physical link to form between them. While exchange of genetic material between the homologs improves diversity among progeny, the primary purpose of crossing-over is to create this physical link between homologous chromosomes as it helps cell division proceed correctly.

What is the general purpose? The purpose of this technique is to identify the general location on a chromosome where recombination occurred during the process of meiotic crossing-over.
Why do we use it? This technique is used to map recombination during meiosis and can answer questions such as:
- How often does a crossover occur on this chromosome?
- How many crossovers on average occur per round of meiosis?
- Which regions of the chromosome are more likely to experience a crossover?
- Are there conditions (e.g. genetic, environmental) where answers to the above questions change?
How does it work?
To figure out where along the chromosome crossing-over occurred in model organisms such as yeast and fruit flies, geneticists make use of recessive, mutant alleles of various genes along the chromosome of interest. Mutant alleles are versions of genes that have a change (mutation) in them that renders them non-functional. Such an allele is said to be recessive if both maternal and paternal copies of the chromosome are required to have the mutant version to create an observable change in the organism. An organism that’s homozygous for a recessive allele will have the mutant version on both chromosomes and will display an observable trait associated with the allele. An organism that’s heterozygous for a recessive allele will have the mutant version on only one chromosome with the other copy having the functional version. Since two copies of the mutant version are required for a recessive allele to cause a visible change in the organism, the heterozygous individual will not display the observable trait associated with the recessive, mutant allele (Fig.1).
To pinpoint where a crossover (shown in Fig. 2) occurred on a chromosome, we can mate an individual heterozygous for a number of recessive mutant alleles with one that’s homozygous for the same alleles. The offspring of this mating will receive one chromosome from the homozygous parent with mutant versions of each gene and one chromosome from the heterozygous parent with functional versions of each gene up to the point on the chromosome where the crossover occurred and mutant versions beyond that. Based on the recessive phenotypes that show up in the offspring, we can determine which two genes the crossover occurred between in the heterozygous parent (Fig. 3).
