by Danica Dy
Technique Name: Lineage Tracing
Fun Rating: 4/5
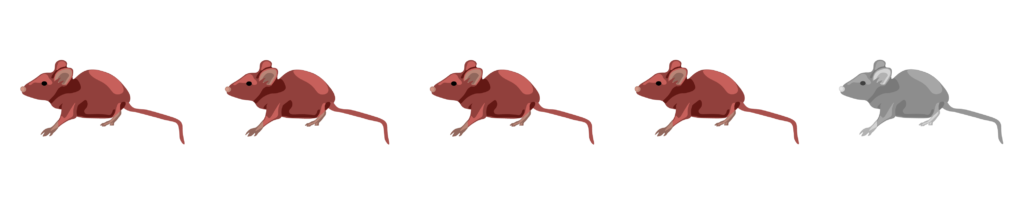
Difficulty Rating: 5/5

What is the general purpose?
Genes provide cells with instructions to make proteins that define a cell’s identity and the functions it performs. When a single cell divides into two cells, the two resulting cells can have diverse gene expression profiles that allow cells to take on different identities and perform different functions. We can label cells before they divide so that the two resulting cells also carry that label. The labeled cells that then divide into four more cells will also carry the label, and so on and so forth. This process is called ‘lineage tracing’. As a result, we can follow the marked cells as they divide to better understand changes in their identity and function, such as those occurring in processes like development and disease.
Why do we use it?
Understanding how a cell’s identity and function change through disease progression allows us to identify interactions between genes driving these changes. Once we can identify which genes may be responsible for cells switching from “healthy” states to “diseased” states, we may develop targeted therapies that prevent, slow, or stop the disease process.
How does it work?
“If you turn into a monster, is it still you inside?” You may recognize this line if you’ve been keeping up with the 2023 series on HBO The Last of Us. In this TV show, following infection with the Cordyceps fungus, the “infected” individuals turn into zombie-like beings. There are different stages and variations to this infection process. Newly infected individuals turn into “runners”. With time, they turn into “stalkers”, then “clickers”, and potentially reach the most advanced stage: “bloaters”. Interestingly, this advanced stage has different variations: “shamblers”, which grew in swampy environments, or the “rat king”, which developed in a hospital. At each stage, the beings become increasingly less like their original human form and more like zombies due to the fungus (Figure 2). This process is similar to the changes a cell undergoes in development or disease, where the cell transitions through different stages that can result in different final outcomes. For example, in heart disease, healthy cells that maintain the heart vessels’ ability to function become injured when disease begins. As a result, the damaged cell can change into other specialized cell types that are not found in healthy heart vessels (Figure 2). Like the “infected”, in a disease process, the once healthy cells become less like their original identity and more like different specialized cell types that are not normally seen in the cell’s environment without the disease.

Lineage tracing can be seen as a tool for answering a similar question, “If a cell becomes ‘diseased’, how did the original cell change?” More importantly, we can trace these changes back several generations of cells to ask “what changes cause disease?” Much like the first patient to be infected with the Cordyceps fungus, we begin with a single cell that divides into other cells, which take on identities and functions that differ from the cell that we started with as disease progresses. Similarly, there are different stages of this transition. “Runners” do not turn into “bloaters” overnight; this process occurs gradually. Similarly, healthy cells in the vessels of your heart undergo gradual genetic changes with disease. A once-healthy cell can later divide to generate cells that build up in the vessel, which blocks blood from passing through. With these disease-related genetic changes, the cell becomes less like its original identity.
Today, scientists can mark a specific population of “healthy” cells in animal models (eg: mice) with lineage tracing, expose the animals to conditions that model disease, and study the tissue from the animal to see how those labeled cells have changed (Figure 3). These lineage-tracing markers are usually fluorescent proteins, meaning that the cells of interest glow under a specific wavelength of light. The three important features of the lineage tracing cell-labeling method are that 1) the marker does not spread to neighboring cells and does not affect them; 2) the marker is passed down to all descendant cells; and 3) the marker glows continuously and does not lose the ability to glow as it is passed on.
This method is particularly important since the trajectory of changes is not always linear. Sometimes a healthy Ellie-like cell may transition into a “clicker” and not undergo further changes. Other times, it may advance into a “bloater”, or with very specific genetic changes, a “shambler”. Labeling these cells with a lineage-tracing marker before they change allows us to identify them even after they’ve turned into something different. Ultimately, we can compare the differences in the genes expressed before and after those changes to identify the responsible genes. Much like developing an anti-Cordyceps vaccine to save the world in The Last of Us, finding ways to target genes responsible for disease progression too will save many lives.